Sharing electronic warfare tasks could revolutionise combat in the electromagnetic spectrum, but just what is distributed EW and how does it work?

The Greek philosopher Aristotle (384-322BC) made an unwittingly prescient observation about electronic warfare when he said, “the whole is something besides the parts.”
Over the intervening 2,370 years since he penned his words in Metaphysics the great man’s words have morphed into the pithier, although misquoted, “the whole is greater than the sum of the parts”.
It may not be immediately apparent as to why Aristotle’s missive is of relevance to EW, but his words are apropos when one considers distributed EW. Distributed EW is founded on the principle of networking disparate EW systems to one another to have an impact at the tactical and operational levels. The rationale behind distributed EW is to provide as detailed a picture of the electromagnetic spectrum as possible in temporal and spatial terms across a specific area.
At first blush, distributed EW may seem a complex concept, yet it rests upon some straightforward principles. Consider a combined theatre-level operation comprising warships, land forces, aircraft, and space assets against a similarly matched adversary. The warships are outfitted with electronic support measures (ESM) which collect communications intelligence (COMINT) on hostile radios and networks, and also collect electronic intelligence (ELINT) on hostile radars – notably those equipping opposing warships and anti-ship missiles.
Combat aircraft above also collect ELINT using their radar warning receivers (RWRs), and some special mission aircraft may have ESM to collect COMINT and ELINT from the ground and maritime environment. The army deploys specialist EW units collecting COMINT on their red forces and may also collect some limited ELINT concerning weapons-locating and ground-based air surveillance radars used by their adversary.
Finally, space continues to grow in its ability to contribute to situational awareness, with signals intelligence (SIGINT) gathering satellites loitering overhead. These gather ELINT and COMINT across the entire theatre. This SIGINT is beamed down to Earth as raw data for analysts within and outside the theatre of operations to interpret.
The joint force commander can receive torrents of information from these myriad sources, and all this SIGINT arrives at their headquarters and is analysed by their EW staff. Relevant information such as the location of hostile radars, the contents of hostile communications traffic, or the location of enemy troops, aircraft, and warships based on their communications and radar emissions, is then shared with deployed units, ships and aircraft.
This intelligence can be exploited to interpret red force intentions, and/or to locate hostile radars, radios, and communications networks. Intelligence can then be transformed into action, with these targets being engaged with cyber, electronic, or kinetic effects as part of the operational and tactical schemes of manoeuvre.

The scenario discussed above is noticeably stove-piped – the SIGINT all arrives at one location, in this case the commander’s headquarters. The EW staff act as a clearing house for this intelligence, with the relevant SIGINT being sent back out to those units, platforms, and personnel who need it. The major shortcoming is the latency, or time lag, between the capture of the SIGINT by deployed sea, land, air, and space assets, and the engagement of a target resulting from this SIGINT.
Distributed EW is a desire to maximise deployed EW assets to support the blue force’s quest for electromagnetic superiority and electromagnetic supremacy through the application of electromagnetic manoeuvre. It is worth looking at this definition in some detail.
Electromagnetic manoeuvre takes the principles of manoeuvre warfare and adapts them to the electromagnetic domain. According to the military theorist and historian Professor Christopher Bellamy, in its purest form, manoeuvre warfare is the use of surprise, deception, and the ability to act faster than the enemy can respond. Electromagnetic manoeuvre views the enemy’s use of the electromagnetic spectrum and applies electronic and occasionally kinetic effects to frustrate the red force’s use of the spectrum.
For instance, friendly forces may discover a hostile ground-based air surveillance radar. This could then be engaged by electronic or cyberattack, which may temporarily or permanently render the radar inoperable. Alternatively, it could be engaged with surface-to-surface or air-to-surface weapons which may destroy the radar outright.
Electromagnetic manoeuvre rests on the ability of friendly forces to navigate Colonel John Boyd’s famed OODA (Observe, Orient, Decide and Act) loop at a faster pace than one’s adversary. Borrowing from the airpower domain, electromagnetic supremacy can be defined as the use of the spectrum by friendly forces at a given time and place of their choosing, without prohibitive hostile interference. Electromagnetic superiority is the necessary precondition to then develop electromagnetic supremacy by which a hostile force is incapable of meaningful interference in the use of the spectrum by friendly forces.
Electromagnetic manoeuvre is arguably restricted by the stove-piped models detailed above. The need to receive SIGINT, analyse this intelligence and then distribute relevant material to friendly platforms, personnel and units slows down the pace at which blue force EW practitioners can orbit the OODA loop. This then places a corresponding restriction on the degree to which electromagnetic superiority can be achieved. Adopting a distributive approach towards electronic warfare is one way in which élan, intrinsic to all manoeuvre warfare, can be enhanced.
DEFINITIONS
With few exceptions, electronic warfare in the sea, land, and air domains has lacked persistence, the exception to this rule being SIGINT gathering satellites in geostationary orbits. These spacecraft have the luxury of loitering permanently above a particular part of the Earth’s surface to continuously ‘hoover up’ signals of interest, and transmit these as raw data back to Earth where they can be analysed.
When electronic warfare began to be used en-masse during World War II, the initial emphasis was on protecting aircraft against detection by radar. As the conflict continued, this developed into using specialist electronic attack planes to supplement the organic EW subsystems carried by individual aircraft for self-protection.
This was best exemplified by the work of the Royal Air Force’s Bomber Command 100 Group which used EW to support the Allied strategic air campaign against Germany. In the air domain, these principles have remained largely unchanged since that conflict, although the technologies to protect individual aircraft and formations have evolved exponentially.

Likewise, the use of EW in the maritime domain has increased significantly since the late 1950s as the advent of the missile changed the face of naval warfare, transforming it into a largely beyond-visual-range contest precipitated by the coming of age of the aircraft carrier. Naval EW is largely focused on the protection of individual ships or task groups against electromagnetic threats, be those from naval surveillance radars equipping warships or the active radar-homing seekers equipping anti-ship missiles.
On land, the collection and exploitation of COMINT to direct kinetic or electronic attack against hostile communications systems and networks, and to support the commander’s scheme of manoeuvre, is the main priority for land forces EW practitioners.
All these scenarios arguably focus on EW being used for the protection of friendly forces and to help them to achieve their mission over a specific timeframe.
That EW is now experiencing a paradigm shift forming the dominant means by which electromagnetic manoeuvre is executed reflects the significance the spectrum has attained for the prosecution of military operations. During World War II, Field Marshal Bernard Montgomery reflected on the importance of air superiority asserting that, “if we lose the war in the air, we lose the war, and we lose it quickly.”
His words are arguably as applicable to the war in the spectrum as they were, and continue to be, for the war in the air. It is almost inconceivable that a near-peer adversary could prevail in battle against the US and her allies without having established a position of electromagnetic supremacy. Likewise, US and allied militaries will need to ensure that they can attain and maintain electromagnetic superiority during any future conflict. Distributed EW will have a key role to play in this regard.
EXAMPLES
While distributed EW may seem a somewhat abstract concept, moves are already afoot to introduce it. “Electronic support is the first cab off the rank here,” Dr Anthony Szabo, information warfare star shot leader in the cyber and electronic warfare division of Australia’s Defence Science and Technology (DST) group told ADBR.
Electronic support forms one of the three pillars of electronic warfare alongside electronic attack and electronic protection: Electronic attack consists of “actions taken to prevent or reduce an enemy’s effective use of the electromagnetic spectrum”, according to US Department of Defense definitions. It says electronic protection focuses on “action taken to protect personnel, facilities and equipment from any effects of friendly or enemy employment of electronic warfare”, consisting of “actions … to search for, intercept, identify, and locate sources of intentional and unintentional radiated electromagnetic energy”.
Dr Szabo says that networking multiple electromagnetic receivers will provide a bigger virtual aperture with which to look at one or more threats in a specific area. In a nutshell, one gets far more detailed information having several eyes looking at the same threat, rather than just one eye staring at it.
Networking these receivers, he argues, provides high angular accuracy of the threat relative to the receivers. This translates into detailed location information on that emitter. The more information that can be derived on an emitter’s location and the characteristics of its transmissions, the more effectively it can be engaged with cyber, electronic, or kinetic means. “These ideas have been developing for some time, and are now maturing,” Dr Szabo told us.
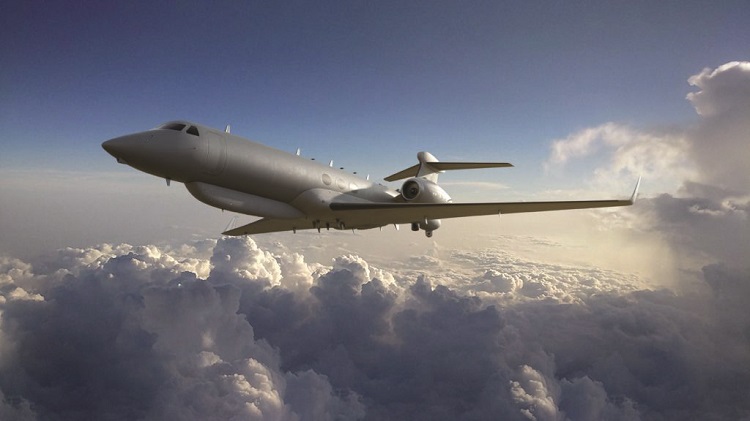
One example of a distributed EW system is NATO’s Cooperative Electronic Support Measure Operations (CESMO) protocol. CESMO provides real-time location information concerning hostile ground-based air surveillance and fire control/ground-controlled interception radars during air operations. The CESMO protocol uses IP (Internet Protocol) messaging to share this information with platforms and personnel involved in the air battle.
The real-time nature of CESMO is a vital attribute, as analysing ELINT collected by an aircraft’s ESM or radar warning receiver after a mission could give those involved in air operations a less-than-accurate depiction of where threats are located. This is because hostile radars – particularly those that are mobile – detected and located during a sortie may have changed locations in the time between the aircraft completing its mission and the ELINT being analysed, thus making the ELINT obsolete by the time it is available for dissemination.
CESMO works to address these shortcomings by gathering and sharing ELINT in real time and, usefully, CESMO does not require a new communications network. Instead, it employs existing ultra-high frequency (UHF – 300MHz to 3GHz) air-to-air radio communications with the relevant data being converted into encrypted IP traffic and sent across UHF networks using a standard modem.
Ioannis Viagis, a lecturer in radar and sensors at Cranfield University in England says that beyond the ESM carried by the aircraft, all that is required for a CESMO network is the aircraft’s standard UHF radio, a cryptographic device to secure the data, and the modem to send and receive CESMO traffic. CESMO takes the ELINT from that gathered by the RWRs and ESMs already equipping NATO combat aircraft.
The location of radar threats is performed using Time Difference of Arrival (TDOA) techniques. TDOA is a triangulation process exploiting the microscopic differences in time it takes the same radar transmission to reach three or more different aircraft positioned at different distances from the radar. Radar transmissions travel at the speed of light so, by computing the individual time taken for the same transmission to reach these aircraft, it is possible to precisely locate the radar.
The Link-16 tactical datalink which is already used by NATO and allied nations for communications in the air domain can already handle some EW information in near real time. But this is primarily restricted to parametric information and electronic warfare control coordination via the J14 and J14.0 series messages carried across this network. Ioannis Viagis says the precision of CESMO is sufficient to pinpoint the location of the emitter to within 500 metres or 1,640 feet.
At the core of the CESMO approach is the VMF (Variable Message Format) protocol which meets the requirements of US Department of Defense Military Standard-188. Viagas says the CESMO software can be installed on a laptop to gather all the lines of bearing to compute the radar’s location.
During air operations, a list of all participating platforms and their ESM/RWR subsystems would initially be drafted. These subsystems would be uploaded with a list of radars that are confidently expected to be encountered in the theatre of operations based on initial SIGINT gathered to support the operation.
As the aircraft perform their missions, they gather ELINT regarding these radars, crucially providing information on their location. The ELINT is sent to the CESMO Fusion and Coordination (CFC) node – a computer hosting the software – which performs the triangulation.
Once this is done, information on the radar’s location is shared with the aircraft involved in the operation. Armed with this information the radar can be avoided or, if this is not possible, attacked. The CFC also processes ELINT delivered across the CESMO network pertaining to new radars discovered as and when they enter theatre using the same identification and triangulation techniques.
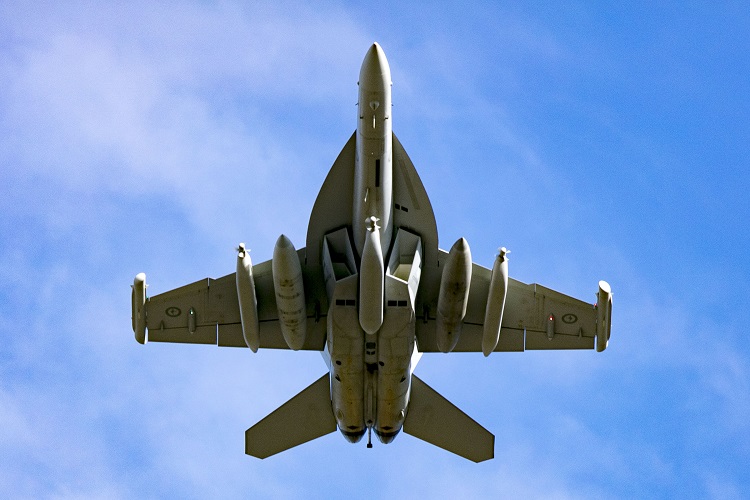
ELECTRONIC ATTACK
Beyond electronic support, Dr Szabo sees clear applications for distributed EW to assist electronic attack. For instance, by networking disparate jammers these systems could attack the same radar or radio from multiple directions. This could go some way towards nullifying electronic counter-countermeasure (ECCM) techniques by which a radar or radio will detect jamming from a certain direction and thus ignore signals coming from that direction.
Jamming from multiple directions may be sufficient to overwhelm these ECCM techniques. “You can produce multiple false targets on the same emitter,” said Dr Szabo. “These ideas have been around for a while. We have done some analysis of techniques, and we are keen to move towards a demonstration of some of those capabilities. When will we have distributed electronic attack? Within a 10-year timeframe I think, but maybe even sooner.”
There are still technological hurdles to overcome. Dr Szabo said that distributed EW techniques could exact a major processing burden on the command and control, and battle management of these networks. “Some nations are seeking to employ Artificial Intelligence (AI) and Machine Learning (ML) to tackle this problem,” he said.
One challenge which will need to be addressed is ensuring that distributed EW systems can prioritise their work accordingly to the most pressing threats and tasks. “How do you prioritise these resources and deal with whichever scenario you are facing?” he asked.
From an electronic support perspective, distributed EW systems may need to use AI and ML to cope with the exponential increase in waveforms today’s and tomorrow’s emitters will have, alongside the ongoing congestion of the electromagnetic spectrum with civilian and commercial communications. “Electromagnetic threats were by and large static emitters that would have a finite number of waveforms,” he said. “Modern threats have much more variability. You might be dealing with emitters with millions of waveforms.”
AI and ML could also make a valuable contribution in helping to search through the flood of clutter collected by a distributed electronic support system to find the all-important signal of interest. Furthermore, Viagas believes that AI and ML will have roles to play in helping to optimise the positioning of ESM to perform the location of hostile emitters ever more accurately.
But he cautions that, for distributed EW approaches to yield results it is imperative that the ESM being used have the requisite processing power, and that communications systems and networks across which the information could be shared can handle the significant quantities of data distributed EW architectures will generate.
This is both from an ESM perspective where huge quantities of relevant data may be coming in from the sensors, and when electronic attack is being performed
Using distributed EW systems to perform electronic attack is likely to require significant ‘fire control’ data on which emitters and are to be attacked and how. During and after the attack, data will likely be generated regarding its course and success, or otherwise. Viagas stresses that low latency when handling high data rates will be intrinsic to these networks operating efficiently.
TRAJECTORIES
The work which has been performed on CESMO indicates the direction of travel for distributed EW, and the likelihood that electronic support may be the first sub-discipline of EW able to exploit these advances. Innovation in the AI and ML sector could spur the development of distributed electronic attack networks, yet such capabilities may still be some way in the future.
While distributed EW is likely to roll out across the land, sea, and air domains, the advent of tomorrow’s 6th generation fighter aircraft like the BAE Systems’ Tempest, USAF’s Next Gen Air Dominance (NGAD), and the Airbus/Dassault Future Combat Air System, will help to accelerate these capabilities. These platforms will be comprehensively networked into their wider environments, and the sharing of electromagnetic data will be paramount. This will help to support electromagnetic manoeuvre and thus assist its kinetic counterpart.
Electromagnetic superiority is no longer optional, and the force which uses distributed EW with imagination and dash is the one most likely to prevail over their adversary.
This article appeared in the September-October 2020 issue of ADBR.